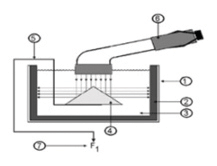
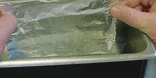
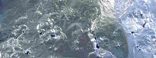
This column is about understanding how and why sonic transducers perform as they do in cleaning operations.
That understanding is based on an analysis of how the power applied from transducers is distributed throughout the cleaning tank. The involved science is sophisticated, but the understanding may come mainly from a simple (and old) method. “BLACK ART” Use of ultrasonic transducers can be as much “black art” as science—both despite and because of the best efforts of suppliers. There are two reasons:
- The technical one is that the continued reflection of pressure waves off tank walls, parts and part baskets, and the fluid-air interface produce a somewhat variable and irreproducible environment.
- The commercial reason is that it is a way of differentiating what has become a commodity product—sonic transducers.
ENTER SCIENCE As does nature abhor a vacuum, so does those valuing science abhor “black art.” This has led over at least the past decade and one-half to many suppliers and users attempting to measure the local effects of pressure waves produced by sonic (ultra and mega) transducers. HIGH-TECH SCIENCE Scientific developments with various levels of success include measurement of:
- Temperature profile throughout a cleaning tank using a polyethylene calorimeter
- Radiation pressure using a velocity sensor
- Local velocity gradients via laser interferometry
- The optical diffraction patterns of short laser pulses due to pressure waves
All of these approaches are useless for cleaning operations. MEDICAL CALIBRATION INSTRUMENTS The mechanical effect of the ultrasonic transducer can be quantified through a radiation force balance. A low-wattage transducer is aimed at a hollow triangular target, as shown in Figure 1. The net applied force causes the target to deflect, and the miro-force required to sustain its position is measured by a strain gage bridge. The gram value of force is directly proportional to the absorbed power level. Cavitation is not involved as the frequencies are megasonic.
These devices that operate in undisturbed tanks are useless for cleaning operations, but do provide good value to sites doing medical work who must calibrate the output of therapeutic ultrasound devices. FIELD-USABLE PROBES Two types dominate attention, but are not all that's available.1,2 NAVAL SCIENCE The first type of these devices are basically microphones (hydrophones)—devices which capture sound energy from pressure waves and convert them to a measurable voltage.
Fortunately, these devices work best with ultrasonic transducers operating at the most commonly used frequencies—40 kHz and 68 kHz; unfortunately, the commercial models don’t work as well with substantially higher frequencies. NON-NAVAL SCIENCE Those which are not based on hydrophones3 evaluate the amount of cavitation energy using both indirect and direct methods. Some methods and apparatuses are claimed in patents; a few others are embodied in commercial products. DIRECT MEASUREMENTS
- Azar in U.S.P. 6,450,184 (2002) claims a single or family of probes dispersed throughout a tank of fluid.
- Azar in U.S.P. 6,890,390 (2005) claims the electronic management of signals produced by the technology earlier patented as U.S.P. 6,450,184.
- Puskas in U.S.P. 6,691,578 (2004) reminds us that an ultrasonic transducer can be either an emitter of radiation (pressure waves), or a receiver of same.
- Kerr, et. al. in U.S.P. 7,111,517 (2006) claims use of a test wafer comprising an array of pressure sensing elements for monitoring power level variation of time-varying pressure waves.
INDIRECT MEASUREMENTS
- Puskas in U.S.P. 6,288,476 (2001) claims insertion of a hollow polypropylene probe into to a working cleaning system.
- The claims by Farrell in U.S.P. 7,057,973 (2006) speak about sonoluminescence—the emission of short bursts of light from imploding bubbles in a liquid when excited by sound.
OLD LOW-TECH SCIENCE Not recently developed, simple, low-tech, and—in my humble view—still the most reliable, is the aluminum foil test.
One simply inserts a supported piece of aluminum foil into the cleaning tank (Figure 2) for 30 seconds to 10 minutes (depending upon the nature of the generated pressure waves), and observes the mechanical damage (peening) upon it.
One may see a slightly dimpled orange-peel pattern. Or one may see the distinctive damage pattern as in Figure 3.
One does this at a selected distribution of locations throughout the cleaning tank (while it is in use doing cleaning work), and compares the observations of foil damage to infer how the cleaning intensity varies within the tank’s contents.
A simple example is where one wraps metal washers with one thickness of aluminum foil and suspends them in the cleaning fluid using wires.
This approach allows (not measures) estimation of what’s wanted to be known—the extent of cavitation damage while cleaning work is being conducted.
However, no data is directly produced (unless weight loss measurements of the foil are made). There is evidence that points of weakness (folds or wrinkles in the foil) act to increase the propensity of the foil to be damaged.
A factor of much lesser concern is that the damage results are not known instantaneously. EXPERIENCE AND OPINION The above has been impersonal, and scientifically inclined. What follows is personal—and experiential.
With clients, and in my own research, I have used two different hydrophone power sensors and one commercial device based on the patents noted above. All were used in actual cleaning operations—both critical and sub-critical—to determine how and why sonic-based cleaning operations were not performing as expected. I also used the aluminum foil test in all situations I believe in it.
I know these instruments can be useful, but I didn’t find their use to add value, beyond the uniform foil test. The former cost several thousands of uniformly; the latter is essentially free.
There are two types of experimental errors: false positives, and false negatives. I found:
- The foil test would not give false positives—if the foil was perforated, it wasn’t done by mice! If local cleaning
- performance was substandard, it was found to be for another reason than ineffective or inefficient cavitation.
- Both types of instruments would give false positives (for whatever reasons)—indications of cavitation activity where cleaning was substandard.
- Both types of instruments and the foil test did not give false negative errors. If the tests suggested cleaning would be poor, because of insufficient cavitation activity, it was observed to be poor.
- Both types of instruments produced results showing a distribution of cavitation activity throughout a filled tank empty of parts, baskets, and other surfaces reflective of pressure waves; free as well of cleaning chemistry and removed soil; and uniformly at the temperature of use. When the tank was put in operation doing cleaning work, that distribution was not found—as expected. The energy distribution was different, at least because of the reflection of internal pressure waves among solid tank components.
SUMMARY Both types of instruments are sure to provide value for some sites in some situations. They wouldn’t exist if they did not. I have no experience of one type of instrument being compared to different type.
I can’t recommend, and don’t use, anything but the aluminum foil test for cavitation intensity in tanks doing cleaning work. This test does not give false positives—mice don’t eat aluminum foil. REFERENCES
- U.S.P. 6,978,677 claims an apparatus for measuring ultrasonic power using an ultrasonic absorber formed from polyurethane. Overlying the absorber is a second membrane polyvinylidene fluoride which acts as a pyroelectric detector (Pyroelectricity is the ability of certain materials to generate an electrical potential when they are heated or cooled). So the effect of addition of ultrasonic energy to water is to raise its local temperature, which is detected and converted to a measurable voltage signal.
- U.S.P. 4,492,107 claims an acoustic power meter with a liquid crystal cell that includes a specific type of liquid crystal material having elongated molecules. The effect of ultrasonic energy upon the liquid crystal material is to change the birefringence of the material, to which an electric field is being applied. The strength of the electric field required to suppress the birefringence is proportional to the acoustic intensity of the ultrasonic beam or acoustic field applied to the cell.
- Azar, L., “Understanding and Evaluating Ultrasonic and Megasonic Cleaners,” Process Cleaning Magazine, Sept/Oct 2007, pages 40–43.
- There is not a one-to-one correlation between cavitation activity and cleaning effectiveness.
BIO John Durkee is the author of the book Management of Industrial Cleaning Technology and Processes, published by Elsevier (ISBN 0-0804-48887). In 2012, Elsevier will publish in print his two landmark books Science and Technology of Cleaning with Solvents [ISBN 9781455731312], and Handbook of Cleaning Solvents, (ISBN-13:978145573144) as well as a 4-part e-Book Design of Solvent Cleaning Equipment. He is an independent consultant specializing in critical and metal cleaning. You can contact him at PO Box 847, Hunt, TX 78024 or 122 Ridge Road West, Hunt, TX 78024; 830-238-7610; Fax 612-677-3170; or jdurkee@precisioncleaning.com.