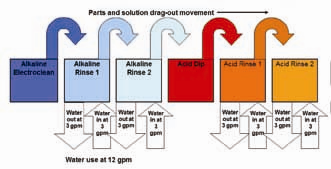
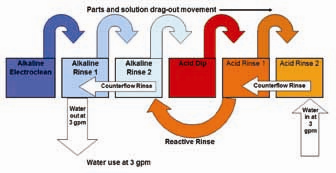
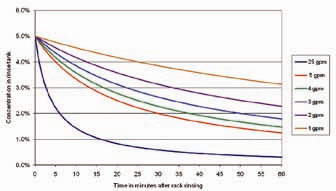
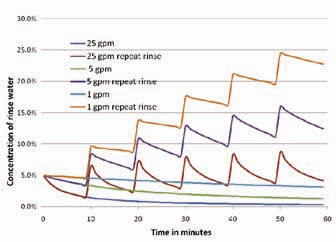
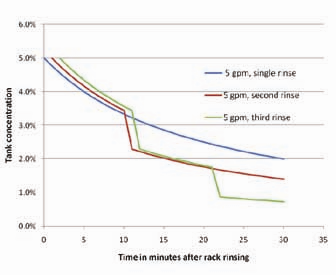
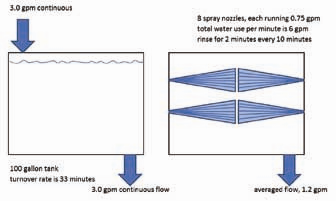
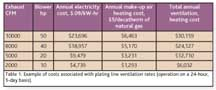
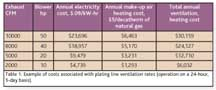
In good economic times, there is not as much motivation for a business to take a hard look at the cost of their plating or finishing processes. With fierce competition from overseas, and a weak economy, the need to look at these processes becomes much more important.
Surprisingly enough, once the real costs associated with plating and finishing lines are known, there are many options available to reduce some or all of those costs, and the economic paybacks can be very short.
We will present the methodology used by the New York State Pollution Prevention Institute at Rochester Institute of Technology to determine the baseline costs of the finishing operation. Potential improvement methods or technologies will be presented for each area typically found in any finishing line.
There are four areas common to almost every plating line and metal finishing line:
- Rinse tanks
- Ventilation systems
- Acid cleaners, acid etches
- Alkaline cleaners
It is important to collect good baseline information on each of these areas. Once that information is collected, it is easy to rank each area by cost and to look for the best options to reduce those costs. It is also very helpful to create a line layout—if one does not already exist—to help clarify the process steps and material flow. As much detail as possible should be contained in the line layout. Decisions will be much easier later in the evaluation if the layout information is complete. A spreadsheet is also helpful to aid in calculating chemical costs, water costs, etc., and can be readily updated as more information is collected.
The following question lists will provide sufficient information to develop baseline cost information.Baseline questions for rinse tanks:Number of rinse tanks after each process tank?What is the rinse tank type (single rinse, reactive rinse, counterflow rinse, stagnant rinse, spray rinse)?What is the flow rate on each rinse tank?What water type is required for each rinse tank (reverse osmosis, deionized water, city water)?Does the rinse water contain either high toxicity or high value material (chromic acid, gold, etc.)?What are the water purchase and sewer costs per 1,000 gallons of water? Note that these water costs can be either combined on one bill or separate. Rochester, N.Y., has monthly water billing and an annual sewer tax based on annual water use.Baseline questions for tank ventilation:How many plating line ventilation systems are there (scrubber, straight exhaust, etc.)?What are the rated CFMs for each exhaust fan?What is the horsepower rating or volt, amp, phase rating for each exhaust motor?What is the total exhaust time per day?What are the heating degree days for your location?What is the building heating (and possibly cooling) cost by month?Are the plating line ventilation systems tied into automatic tank covers?Baseline questions for the acid and alkaline tanks:What are the tank volumes?What is the tank chemistry concentration?Cost of the chemistry per tank refill?Tank dumps per year, and reason for tank dumps?Cost to treat the chemistry after the tank dump (labor, neutralization chemicals, sludge disposal, etc.)?Once armed with the baseline information, it is relatively easy to determine the real cost for each area. Then it is possible to prioritize the costs and target cost reduction changes. The following baseline example is from a medium-sized job shop plating company that was part of a direct assistance program through the New York State Pollution Prevention Institute. The baseline list has been ranked by cost.
- Water use = 6,310,000 gpy (gallons per year) = $32,900/yr. ($5.22/1000 gallons)
- Acid purchases (HCl) = $19,700 ($1.25 /gallon, 15,760 gallons)
- Waste treatment sludge disposal = $15,600/year
- Exhaust blower = 10,000 cfm = $7,899/yr. for 40 hours per week ($.09/kwh)
- 5. Caustic purchases (NaOH) = $6,400 ($2.10/lb, 3,048 lbs.)
- Heating of make-up air = 431 decatherms = $2,154 /yr. ($5/decatherm for natural gas) Total cost per year = $66,923/year
In this example, the water cost was by far the highest single cost to the company for their plating lines. A close second and third were the acid purchases (included line acid and waste treatment acid), and waste treatment sludge disposal.RINSE WATER OPTIONSIt might not be typical for all metal finishing operations but it is fairly common to have water costs at or near the top of the cost of operations. Rinsing is critical in the metal finishing process, but more water use does not necessarily mean better rinsing.Best practices for producing effective rinsing are:
- Multiple counterflowing immersion rinse tanks between process tanks
- Reactive rinsing for the appropriate process chemistry combinations
- Spray rinsing
- Combination rinses such as immersion rinsing, followed by spray rinsing or reactive rinsing combined with counterflow rinsing
Figure 1 shows a rinse tank system with multiple rinses but with no counterflow rinsing on any of the neighboring rinse tanks. In this example, if each rinse tank is a flowing rinse, the total water use is 12 gallons per minute (gpm)
There are two means of reducing the water use in rinsing without reducing the flow rate in each tank. The first is called counterflow or countercurrent rinsing, where the relatively clean rinse water from the second rinse in a rinse tank pair is flowed to the more contaminated primary rinse tank. Therefore, cleaner water is always moving to less clean rinse tanks. The cleanest water is still used for the critical final rinse, but the same rinse water is reused for the initial and least critical rinse. In Figure 2, if counterflow rinsing was the only additional water-saving method used, there would be a 50% reduction in water use (6 gpm) compared to Figure 1 (12 gpm).
The second, less commonly used method of reducing water use is called reactive rinsing. It is a method of taking rinse water around a process tank to a previous rinse tank. The example in Figure 2 shows acid rinse water (acid rinse 1) flowing to the last alkaline rinse tank (alkaline rinse 2). The acid contained in this rinse water would normally be sent to waste treatment. With reactive rinsing, the acid from acid rinse 1 now goes to alkaline rinse 2 and neutralizes the residual alkalinity in that water. Any rinse water from alkaline rinse 2 being dragged out by parts and racks to the acid tank will now contain acid which previously would have been wasted. Therefore, no acid is being neutralized by alkaline dragout to the acid tank, and acid previously lost in acid rinse 1 now has some recovery by the reactive rinse flow. Figure 2 has a total water use of 3 gpm compared to the original flow rate of 12 gpm. The cost savings is $5,400 per year at $5/1,000 gallons for an 8-hour-per-day, 50- week operation, if 9 gpm is saved.
Oftentimes, when the rinse appears to be inadequate, companies assume that the best method of improvingan immersion rinse is to increase the flow rate. However, rinse flow rates can be deceptive in that high flow rates might not be as helpful as expected. Figure 3 displays rinse tank concentration over time at various flow rates. The initial conditions are: 100 gallon rinse tank, incoming (dragout) solution concentration of 100 grams/gallon, and a dragout volume per rack of 0.05 gallons. It is apparent from Figure 3 that the rinse tank does not dilute the dragged-in chemical very rapidly. Even the 25 gpm flow rate takes approximately 5 minutes to drop the concentration from 5% to 2.5%. The main point is that a single rinse tank is relatively ineffective at providing critical rinsing. More importantly, increasing the flow rate in a rinse tank does not necessarily improve rinsing unless extremely high and costly flow rates are used.
By taking immersion rinse flow rates one step further, Figure 4 shows the same rinsing example as shown in Figure 3, with the exception that every 10 minutes an additional load of dragout chemical is added. Note that this causes the rinse tank concentration to rise to very high concentrations very quickly, regardless of the flow rates used. This is another reason that counterflow rinsing is so effective. The concentration of the dragout chemistry between the first rinse tank and the second rinse tank drops dramatically. Thus, the effective dilution rate due to water flow is much faster, as shown in Figure 5.
The final method of reducing rinse water volumes but still obtaining excellent rinsing is by spray rinsing. This method is somewhat limited by the geometry of the parts being rinsed in that complex geometric shapes are difficult to thoroughly rinse with an automatic spray system. In a manual line, the operator can overcome the geometry problem of a part by manually spraying the part areas that are difficult to rinse by a normal battery of spray nozzles. Figure 6 compares a spray rinse to an immersion rinse. There are two major advantages to spray rinsing over immersion rinsing. First, the water hitting the parts is always clean—unlike water in an immersion tank which always contains some residual contamination. Second, a spray rinse needs to be running water only when parts are being rinsed. The rest of the time there is no water use, which is both a cost and environmental savings. A third and lesser advantage to spray rinsing would be in the case of parts requiring a heated rinse. In-line demand heaters can be used to provide hot water as needed during the spray cycle rather than having to continuously heat an immersion rinse tank.
The spray system in Figure 6 illustrates the water savings associated with spray rinsing compared to immersion rinsing. The left illustration in Figure 6 is a typical immersion rinse tank running at 3 gpm. The right illustration is a spray rinse with a battery of eight spray nozzles with a combined spray volume of 6 gpm. The spray rinse in this scenario is only turned on for two minutes while parts are in the tank. The next set of parts arrives eight minutes later. Since the spray rinse is turned on only for two minutes out of a 10 minute period, the average water use is 1.2 gpm, which is less than half of the immersion rinse tank’s usage rate of 3 gpm.
One final way to reduce rinse water use in immersion rinse tanks is by controlling the rinse water valves. This method is a means of limiting flow when rinse water control consists of manually operated valves. The simplest method is to insert flow restrictors on the water valves to limit the maximum flow regardless of the valve’s position.
Another method of water valve control is to insert solenoid valves into the rinse water lines which open or close based on the conductivity of the rinse water in the tanks. This requires minor up-front measurements of the water conductivity, which is often directly related to the amount of chemistry being dragged into the rinse water. The valve conductivity controls are then set to turn the water on when the conductivity (contamination) gets too high and then turn the water off when the conductivity drops to a lower set point. The advantage of this system is that the water stops running when a plating line has a break in the work flow, rather than manually turning the water on and off at both the beginning and end of the day regardless of the amount of work running through the line. These conductivity controlled valves can be purchased as systems that include the solenoid valve, conductivity probe, and conductivity control box, and typically cost between $500 and $1,000 (Myron L Company).Water Reuse:Most metal finishing industries have in-house wastewater treatment to economically dispose of the acids, alkali, oils, and dissolved metals in the rinse water and occasional tank disposal. However, after treatment this water is typically sent to the sewer since there are still chemicals in the water which makes it unsuitable for reuse. The main post-treatment chemicals in the water are salts such as sodium chloride from the neutralization of hydrochloric acid and sodium hydroxide. Other residual chemicals could include soaps, chelating agents, or surfactants which would be problematic in recycled rinse water.
Typical treated wastewater is:
- Very low in dissolved metals
- Very high in total dissolved solids (TDS) from neutralization and treatment
- Consistent pH, typically slightly alkaline from metal precipitation process
- At room temperature
- Often mixed with residuals such as oils, soaps, or emulsifiers
Both money and labor were spent to treat this wastewater and money was spent to purchase the water and send it to the sewer. Therefore, reusing the water in the process is a means of recovering a portion of that cost. A reverse osmosis (RO) system is one means of recovering at least 50% of this treated water and making it very useable as rinse water again. Reverse osmosis is a technology that filters water with a membrane and allows only water molecules and small amounts of sodium, chloride, or potassium to pass through the membrane (0.5 to 3% leakage of salts is typical). The actual process works by applying pressure to the “dirty” water, which forces the clean water through the membrane and leaves the larger molecules behind.Advantages of RO filtration:
- Removes everything: ions*, bacteria, viruses, solids, dissolved solids
- Relatively simple, low maintenance system
Disadvantages of RO Filtration:
- Low temperature water produces lower pure water yields
- Higher TDS water produces lower pure water yields
- Tends to leak small amounts of single charge ions (Na+, K+, Cl-)
- Membrane can foul rapidly if suspended solids are high (may require pre-filtration with an ultrafilter)
- The RO process is relatively slow such that the most economical RO unit will be running during both production and non-production hours (filtering stored treated wastewater and storing filtered water during off hours)
Current technologies allow up to about 75% fresh water yields. More typical yields are 50% at optimum conditions of temperature and minimal TDS levels. Even with recovery rates of 50%, typical RO systems have a payback of one to two years with water savings. As an example from a case study, an RO unit rated for 15,000 gallons per day water recovery would cost approximately $20,000 and save approximately 3.2 million gallons per year ($17,000 savings/year).
Before purchasing an RO system, it is important to implement other water savings measures first so that the RO system is properly sized for the reduced water volumes. Otherwise, the RO system will be underutilized as other water savings measures are implemented.EXHAUST SYSTEMSExhaust systems are an essential part of the plating line designed to remove dangerous fumes from the process tanks. Typically the highest cost of an exhaust system is the electricity used to run the exhaust blowers. The secondary cost will be very location-dependent and is the cost of reconditioning the make-up air either by heating, cooling, or both. As noted in the plating company example referenced above, the cost to run the blower was roughly four times the cost to heat the make-up air in the upstate New York climate. If the total exhaust requirements can be reduced, then the exhaust blower will be smaller and the make-up air costs will be proportionally smaller. Table 1 shows the total costs associated with various size exhaust systems as the system size changes. Average heating-degree days in Rochester, N.Y., were used to determine the heating costs.
One method used to reduce the total exhaust requirements is with automatic tank covers and variable speed fan controls within the exhaust system. If only one tank requiring exhaust is open at a time, then the exhaust system size can be reduced to handle the full required CFMs for that tank and some small additional CFMs to provide fume extraction from under the closed tank covers. Unfortunately, the best time to implement this technique is on a new plating line. Retrofitting an existing line is sometimes possible depending on the type of the line, but is likely to be more expensive than incorporating this type of system into a new line. As can be seen from Table 1, the cost savings can be significant, even if the exhaust system is reduced by only 50%.ACID CLEANERS AND ACID ETCHANT LIFE EXTENSIONThe starting point for extending the life of an acid bath is having good process controls for the acid bath. Without good monitoring and acid addition methodology, an acid bath can be prematurely disposed of just because the acid strength was not kept at the proper level. If an acid tank is minimally managed, such as running a tank for a month and then disposing of it with no acid additions or titrations over that time period, then the tank effectiveness is variable and unknown. This could lead to plating or finishing defects as the tank ages. If the tank, in reality, was in good condition in that time period, then disposing of the tank is a needless waste of acid and an added cost to treat the acid waste. In another direct assistance project, the New York State Pollution Prevention Institute was able to reduce a 500-ton-per-year acid waste stream to a 250-ton-per-year waste stream at a savings of almost $200,000 per year. Rigorous acid management practices were used to produce these savings.
Good process control means that there is a routine sampling of each acid tank for chemical analysis. On a weekly basis, and in the case of high production lines, a daily titration of the acid baths may be necessary to properly control the acid strength. Then there should be equally regular acid additions to the acid tanks based on the titration results to bring the acid levels back to their original strengths. For large operations there are systems available that do the titrations and acid additions automatically, such as Scanacon titration and acid-dosing equipment.
Second, and usually less frequently, each acid tank should be measured for dissolved metal content. These two tests, titration and metal analysis, are the basic requirements for the proper function of the acid process.The main reason to dispose of an acid tank and start with a fresh chemistry is due to dissolved metal concentrations being high enough to interfere with the acid-metal reaction. Therefore, a means of extending the bath life involves either removing the dissolved metal or converting the dissolved metal to a form that no longer interferes with the acid-metal reaction.
There are three commercially available methods that deal with the dissolved metal problem.1
- Additives to precipitate and/or sequester the dissolved metal
- Diffusion dialysis
- Acid sorption
1. AdditivesMetal precipitation/sequestering is an in-tank means of removing a portion of dissolved metal by precipitation and a portion by sequestering (possibly chelation). PRO-pHx™ (www.pro-phx.com) is one example of such a chemical method. PRO-pHx has a proprietary formulation, but it is believed that part of the chemical reaction produced by PRO-pHx™ involves metal being sequestered because dissolved metal concentrations can go much higher than what would be expected without any apparent loss of acid-metal activity. The high concentrations of dissolved metal are prevented from interfering with the normal acid-metal activity which would indicate some form of sequestering action.
In normal operating use, PRO-pHx is added to the acid tank to maintain a 1% concentration of the additive. A portion of the dissolved metal forms a precipitate that can be filtered. The remainder of the dissolved metal stays in the acid tank but in a form that is not active.2. Diffusion dialysisThe diffusion dialysis process makes use of a membrane that allows the acid’s negative ions (SO4-2, NO3-2, Cl-1, etc.) to pass through while preventing the positive metal ions from passing through. A typical system is 90% efficient, meaning that 90% of the acid is recovered and 90% of the metal is removed in each membrane pass. The results are a waste stream that is high in dissolved metal and a acid stream that can be returned to the acid tank.3. Acid sorptionThe process of acid sorption works on the same principle as ion exchange in a water deionization system. The acid anions (negative charge) are captured from the acid solution stream by an ion exchange resin while allowing the positive metal ions to pass through. Then the resin column is back-flushed with fresh water to free the acid anions. This back-flushed solution is, therefore, rich in acid and poor in dissolved metal. The acid-rich solution can then be returned to the acid tank. This method is between 80% and 90% efficient.
The acid sorption process is commonly used in large aluminum anodizing systems to maintain the amount of dissolved aluminum in the correct range.
The economics will determine which method of acid recovery makes sense for each metal finisher. Again, that is why it is critical to know the cost of acid purchases and disposal to determine the payback for acid recovery systems.ALKALINE CLEANER CONTROL AND LIFE EXTENSIONIn the typical metal finishing process, the alkaline cleaning tanks are first in line and take the bulk of the dirt load. Whether the tanks are soak, ultrasonic, or electrocleaners, their purpose is to remove oils, grease, wax, polishing compound, particulates, and light oxides from the part surfaces. Depending on the detergent additives in these tanks, the tanks could build up surface oil, oil emulsions, suspended solids, or sludge at the bottom of the tank or any combination of these contaminant types. As with acids, the cleaning chemicals are consumed in the process of removing and preventing redeposition of the contaminants.
First, there should be a procedure in place to monitor the alkaline cleaning strength of a bath. It may be as simple as measuring the pH. Typically the cleaning chemistry supplier can either do the testing or provide test kits or test methods to monitor and correct the cleaning chemistry as it ages.
Second, the surface oils can be segregated and removed by a combination of surface sparging and the use of various oil skimmers available on the market.
Third, the heavy particles that can settle on the bottom of the tank can be removed by bag filtration or some other simple filtration method.
Finally, there are the emulsified oils and suspended solids. These are more difficult to remove by normal filtration methods. Ultrafiltration is a method that can often break the oil emulsions and remove the suspended solids without removing the active cleaning chemistry. Some of the commercially available ultrafiltration systems can handle pH from 2 to 11 and temperatures up to 160°F. One unique ultrafiltration system manufactured by Arbortech Corporation has filtration capability of a 1 to 14 pH range and temperature limits of over 200°F. Therefore, this system can easily filter hot alkaline cleaners without filter damage. By whatever ultrafiltration method used, the resulting filtered cleaning solution should have minimal loss of the cleaning chemistry and maximum removal of the suspended solids and emulsified oils such that the cleaning chemistry is ready to use again.
Again, the economics of the cleaning process will drive the decision- making process. If the cleaning chemicals are inexpensive and easy to treat in wastewater treatment, and if tank life is already extended before contamination levels become excessive, then only the simplest and least expensive methods need to be used to provide acceptable cleaning chemistry maintenance.
SummaryIn conclusion, there is often a large opportunity for plating industries to reduce their costs, minimize their environmental footprint and remain competitive in their sector by various relatively simple and sometimes low- cost process changes. By developing a baseline for the energy use, chemical use, and water use for the process, a list of priority focus areas will be determined and the opportunities for cost savings will become evident. In regards to the finishing line, an essential first step is to develop a set of best practices for rinsing and rinse control for water use optimization, along with good process control for the acids and alkaline cleaners. By understanding the overall detailed costs of the metal finishing process, decisions can be made to determine where the major opportunities are and implement changes that financially benefit the bottom line. BIO Dave Fister is a senior staff engineer at the New York State Pollution Prevention Institute (NYSP2I) at Rochester Institute of Technology. He gained 17 years’ manufacturing experience in light industry before he joined RIT. He worked for four years in Manufacturing Technology at Eastman Kodak and 13 years at Bausch & Lomb in various areas of manufacturing and research. During his tenure in industry, Mr. Fister developed strong technical skills, including in-depth knowledge of failure analysis, friction and wear, plating and finishing, joining technologies, fixture and gage design, and metal injection molding. Mr. Fister holds a BS in biology from Cornell University and an MS in materials science from Vanderbilt University.