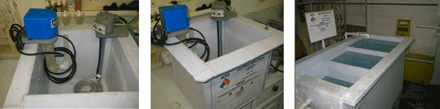
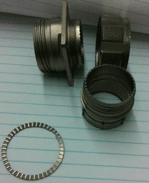
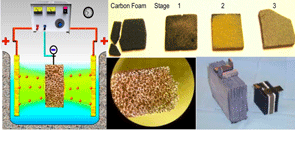
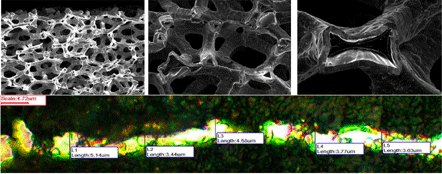
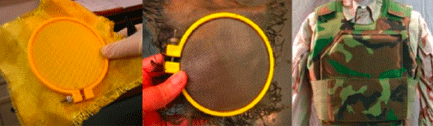
Electroplating of titanium is commonly considered as an “impossible” process. It is known that it is almost impossible to electrodeposit titanium from aqueous solution because of its large negative redox potential [1]. The standard electrode potentials of titanium and zirconium are much more negative than that of hydrogen [2], which would result in reduction of gaseous hydrogen.
Titanium is a metal characterized by a low density (4.5 g/cm3), high tensile strength, and resistance to fatigue, cracking, and corrosion. It is resistant to lengthy exposure to seawater, aqua regia and chlorine. Titanium was discovered in the last decade of the 18th century, and its most common compound, titanium dioxide, had found immediate applications in manufacturing of white pigments (titanium white). Besides that, the main applications of titanium and its alloys are in aerospace (aircraft, spacecrafts, armor plating, missiles), marine industries (ships), automotive industry (high-end products), for production of corrosion-resistant pipes, sport equipment (tennis rackets, golf clubs, cricket, bicycles), as well as medical equipment and products (orthopedic equipment, implants, joint replacements, and surgical equipment). The big advantage of titanium is that it is non-toxic and non-allergenic. Titanium and titanium-zirconium alloys offer excellent corrosion resistance, as they form a strong passive film on their surfaces.
Since production of the metallic form of titanium is a complex and expensive process, we propose to develop an electroplating technique for coating various materials, including plastic, composite materials and carbon foam, with titanium or titanium alloys. The main idea behind that invention is to design new kinds of materials that will: a) have lower cost compared to metallic titanium; b) maintain the tensile strength, corrosion resistance and other properties of titanium metal; and c) be lightweight. This is achieved by combining already low-density plastics, or carbon foam with electrodeposited light titanium metal or titanium alloys. The densities of most popular plastics are in the range from 0.9 to 2.2 g/cm3. This density will be only slightly higher for materials composed of plastic or carbon foam electroplated with thin layer of titanium or titanium alloys.
An inexpensive and simple commercial titanium coating method is not presently available. The only used methods of vapor deposition or titanium cladding is a very expensive process (Modern Electroplating, p.497). Several methods of electroplating titanium on metals have been proposed by academic groups, but none have been incorporated into larger scale commercial production. These methods include: electroplating titanium on iron by galvanic contact in NaCl -TiCl2 molten salt [3]; electrodeposition of titanium from TiCl4 in the ionic liquids at room temperature under argon atmosphere [3-4]; electrodeposition of Al-Ti alloys from the Lewis Acid from melted salt [5], or utilization of a disproportionation reaction of Ti2+ to metallic titanium and Ti3+ on iron in molten salts at high temperatures [6-9]. The Process and Initial Results. We proposed and developed a method for electroplating titanium alloys in the form of a coating on plastic and carbon foams, and to develop reproducible and robust scaled up approach for commercial applications. The reduction of titanium and zirconium before hydrogen will be carried out in a diluted sulfuric acid solution, whose pH will be maintained at the value of ~5 by using an appropriate buffer. At this pH value, the reduction potential of titanium ions shifts toward more positive values. This effect has been demonstrated by the polarization curves of the Ti–Zr system that was potentiodynamically measured in the sulphuric acid solution [10]. This behavior can be demonstrated using experimentally determined Pourbaix diagram [11] that represents dependence of the reduction potential with change in pH (a potential/pH diagram). The diagram shows the mapping of possible stable phases of an aqueous electrochemical system in various pH conditions. In our case, when the pH of the electrolyte is maintained at ~5, titanium is in the form of Ti02.
The initial steps involving surface activation and nickel plating were achieved without any complications. On the other hand, the process of titanium plating was difficult because the plating solution forms a very heavy suspension. We experimented not only with the composition of the initial solution but also with the additives to increase and stabilize the conductivity.
In our first attempts we experimented with the composition of the plating bath, and we tried to plate several materials. During that development, the following elements were taken into account: 1) solubility of K2TiO3; 2) concentration of the final solvents obtained from initial highly concentrated (98%) sulfuric acids; 3) the pH of the plating solution (pH ~5) and a method of maintaining it; and 4) additives to the plating bath.
In our experimental set-up, the oxidation reaction occurs at the anode, which is made of platinum/titanium. Titanium ions Ti2+ move toward the cathode, where they are reduced and deposited as a metallic Ti. This process needs to occur before reduction of hydrogen ions. Our cathode is a nickel-plated over ABS/PC plastic or carbon foam element. Nickel acts as the cathode because it has been passivated and thus electrochemically inactive. In an electrolytic process, negative charges from a power supply are provided to the cathode where they reduce incoming ions. Passivated nickel cannot participate in near-electrode processes, thus only titanium or titanium/zirconium ions are reduced on the cathode, forming an amorphous layer.
Two methods of titanium deposition were designed and optimized. One was a conventional electroplating method—which used constant current—and another one was a nanocrystalline electrodeposition (we refer to as the “turbo method”), which uses pulse plating. In both methods electrodeposition of the titanium alloys proceeded from the sulfuric acid solution. Nanocrystalline electrodeposition (turbo) produces fine-grained coating with significantly improved mechanical and chemical properties compared to conventional constant current plating.
Conventional plating produces larger sizes of grain deposits. The electrodeposited layer is a thick (>20000 nm); fine-grained coating (the average grain size is 6 nm–10,000 nm of metal alloy) of high resilience (>250 MPa), deposited at high rates (>2 μm/hr). Figure 3 demonstrates the difference between the two methods of metal electrodeposition. In the conventional method the size of the crystalline grains is in the order of 5,000-50,000 nm; therefore, our result remains in the nanotechnology field where the customary size of fine grains is in the range 10-10,000 nm.
The methodology proposed here will allow for general industrial applications of the electroplating technology for depositing the titanium–zirconium coating on ABS or ABS/PC plastics and other substrates, including carbon foam. Our goal is to design materials that will be used for: new anticorrosive surfaces, new lightweight materials for air and transportation industries, or electrodes for new generation of batteries. So far, such technology has not been commercially available. We estimated that the cost of products manufactured from titanium deposited on plastic or carbon foam, a method invented by 3C (Crista Chemical Company), would be significantly lower than the currently available products that use Ti metal or its alloys. In mass production that cost could be even an order of magnitude lower. We believe it will lead to highly innovative, lightweight, long-living and green products.
Products. Our goal was to develop a novel, low-cost, industrial method of plating titanium and titanium-zirconium on ABS and ABS/PC, Ultem polymers, carbon foam and other metallic and nonmetallic materials. Materials covered with Ti layer radically gain strength and, for example, shear strength of Ultem plastic becomes comparable to stainless steel. Another dramatic improvement is resistance to corrosion. One of these novel materials, carbon foam, will be a basis for development of new electrodes for batteries.
The market for small parts components for the defense and air industries is quite substantial. It is estimated that in excess of $3.5B is spent annually on replacement connectors. The routine maintenance needed for the connectors exposed to the influence of elements is a significant problem. We estimate that around 10% of the total connector market is in need for the more permanent solutions against the corrosion. This leaves the accessible connector market at $400M.
The first products, the Ti-covered aluminum connectors, were successfully produced using our technology, and at present, they are undergoing the corrosion testing. This product was developed in collaboration with our partner, CalTech Plating. The bulk of their business is in plating small parts, including the connectors. In collaboration with them we have developed a prototype capable of withstanding the specification of 2000 hours of salt spray requirements. We present an example of one of the connectors prepared for CalTech plating that was covered with 20 microns of Ni and 4 microns of Ti. (See Figure2).
We have to optimize the process whereby the connectors would be able to pass stringent corrosion testing (minimum of 2000 h) and subsequent milestone would be the approval of the technical specifications. This pathway to the market provides a significantly lower risk of reaching the level of sustainable revenues needed for the development of other products.
At present, Crista Chemical Company is working on developing the replacement for the existing lead-acid battery with the electrodes constituted from carbon foam plated with titanium. There are numerous advantages of this technology, such as: small size, increased capacity, fast recharge, high- and low-temperature resistance, full recovery after the discharge, resistance to vibration.
One of the most important and required characteristics of the electrodes is their large surface areas. This is achieved by employing highly porous materials such as carbon foam for the basic structure of the electrodes. Our two-stage (nickel and then titanium) plating process provides necessary metal components for conductivity and strength, maintaining at the same time very low weight, compared to lead based batteries and providing high power capacity.
We have already proven the feasibility of plating the carbon foam with Ni and Ti. Electrochemical potential indicates that Ti electrodes may provide several advantages over existing designs. It will provide a durable battery cell with ~2 V of electromotive force comparable to the traditional lead acid battery, but providing more load that would be proportional to the active surface are of the electrode. Approximately 30 times larger surface would result in an order of magnitude increase in potential charge and consequently lowering the size. Because the foam material would be significantly lower in weight overall advantage would be measured by increased charge with decreased weight, volume (see Table 1).
With the advent of electric cars there is a heightened need for better energy storage devices. Because this market is already ~$30B, even with a small size of addressable market ~10% the market opportunity can become significant especially with a novel and important product.
The main barrier would be to create a reliable, single electrochemical cell that would withstand around 500 cycles of recharging. Upon achieving this milestone we could form a partnership with a larger battery producer to rely on the partner to market our components to major battery manufacturers.
Recently we have turned our attention to two additional products. The wheelchair industry has a significant potential for material replacement. If plastic pipes could attain the mechanical properties of steel or titanium metal, then it would be feasible to proceed with a cheaper and potentially easier material. We have succeeded in activating the surfaces of ABS and PC/ABS plastic pipes. The process successfully deposited around 800 min of metal surface. The pipes are undergoing the mechanical testing.
Finally, we tried to test our methodology on a material that might become the technology of the future for personal protection industry. We have successfully activated and Ti-covered Kevlar—an organic material with extra strength that is used for the production of helmets and bullet-proof vests (see Figure 5). CONCLUSION The founders of Crista Chemical Company (3C) strongly believe that creation of this new platform technology will lead to the emergence of many new materials that will find use in a variety of industries. Crista Chemical Company has delivered the proof of concept and now is ready to move to the pilot and production phases. We are open to form a joint venture with an appropriate partner or for other investment options. For more information, please contact Dr. Bog Stec directly at bog.stec@cristachemicalco.us or Robert Krasuski at robert.krasuski@cristachemicalco.us for business development matters. Please e-mail margaret.parker@cristachemicalco.us for all questions related to the technology.
Carbon Foam Ti |
Lead Acid | NiCd | NiMH | NiZn | Li-ion | |
Cell Voltage (V) | 2.3 | 2.1 | 1.2 | 1.2 | 1.4 | 3.6 |
Energy/Weight | 20x | 1x | 1.3x | 2x | 1x | 3x |
Energy/Volume | 5x | 1x | 1.4x | 2.5x | 2x | 3.5x |
Active Surface | 30x | 1x | 2x | 7x | 7x | 6x |
Charging Cycles | 3000 | 500 | 1500 | 500 | 500 | 100 |
Eco-Friendly | Yes | No | No | Yes | Yes | No |
Price/energy unit | 0.3x | 1x | 2x | 0.7x | 0.8x | 4x |
ABOUT 3C TECHNOLOGY Titanium electroplating technology was developed by the group of Engineers and Ph.Ds scientists of Crista Chemical Company LLC. (2010-2011) The business – Crista Chemical Company LLC was primary established in 1999 at Farmington Hills, Mich., where the original concept and the formula of titanium plating was first invented by Margaret Parker, the founder of 3C. Parker has hands-on experience and scientific knowledge in electrochemistry (MS) as well as in organizing and building electroplating lines in commercial environment/set-ups at major U.S. companies. The founder designed and developed many new plating lines for microchips-electronics and automotive industries. She is an expert in optimizing plating processes with application of 6 SIGMA and Statistical method (SPC) principles. Parker graduated from University of Michigan, Ann Arbor, with an MBA/MS degree of Engineering Management and recently completed Six Sigma Black Belt Certification. The other members of the organization are highly educated (PhD’s) with over 15 years of experience on average at the university and business environment.
REFERENCES
1. Gray AG, editor,. Modern Electroplating. New York: Wiley; 1953. 2. Uda T, Okabe, T.H., Waseda, Y., Awakura, Y. Electroplating of titanium on iron by galvanic contact deposition in NaCl-TiCl2 molten salt. Science and Technology of Advanced Materials. 2006;7:490-5. 3. Mukhopadhyay I, Aravinda, C.L., Borissov, D., Freyland, W. Electrodeposition of Ti from TiCl4 in the ionic liquid 1-methyl-3-butyl-imidazolium bi (trifluoro methyl sulfone) imide at room temperature: study on phase formation by in situ electrochemical scanning tunneling microscopy. Electrochimica Acta. 2005;50:1275-81. 4. Mukhopadhyay I, Freyland, W. Electrodeposition of Ti Nanowires on Highly Oriented Pyrolytic Graphite from and Ionic Liquid at Room Temperature. Langmuir. 2003;19:1951-3. 5. Tsuda T, Hussey, C.L., Stafford, G.R., Bonevich, J.E. Electrochemistry of Titanium and the Electrodeposition of Al-Ti Alloys in the Lewis Acidic Aluminum Chloride-1-Ethyl-3-methylimidazolium Chloride Melt. Journal of The Electrochemical Society. 2003;150(4):C234-C43. 6. Straumani ME, Shin, S.T., Schlechten, A.W. JElectrochem, Soc,. 1957;104:17. 7. Steinman JB, Warnock, R.V., Root, C.G. JElectrochemSoc,. 1967;114:1018. 8. Oki T, Choh, T. Bull Jpn Inst Metals. 1983;22:723. 9. Muro M, Takenaka, T., Mawakami, M. Collected abstracts of the 2005 autumn meeting of the Japan Institute of Metals. 2005;137:526. 10. Greenwood NNE, A. Chemistry of the Elements. 2nd ed. Oxford: Butterworth-Heinemann; 1997. 11. Pourbaix M. Atlas of Electrochemical Equilibria in Aqueous Solutions Houston, Texas; Cebelcor, Brussels: NACE International; 1966. 12. Osero NM. An Overview of Pulse Plating. Plating and Surface Finishing, 1986.