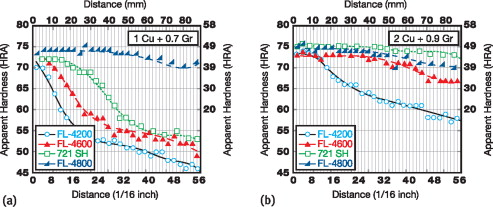
The exaggerated volatility in energy and raw materials prices over the last few years has resulted in continuing problems for component manufacturers in all industry sectors. For ferrous powder producers as well as PM parts makers, “riding the alloy price tiger” has caused a flurry of development activity aimed at finding lower-cost solutions of various kinds, particularly for high-performance applications. As expected, there was no shortage of presentations at the MPIF's PowderMet2009 conference in Las Vegas discussing various aspects of leaner alloy systems for high-strength PM steel components.
Much of the debate so far has ranged around finding satisfactory alternatives to low-alloy steel compositions with high levels of the traditional elements nickel, copper and molybdenum that saw extraordinary price rises up to the current recession. As is well known, these alloy systems have been popular for a long time because of their ease of application in the conventional PM process. Nickel and copper are typically used in elemental admixed form, while molybdenum has found increased application as a fully pre-alloyed constituent because of its strong contribution to hardenability and minimal impact on compressibility. All three elements have the advantage of easily-reducible oxides which makes them very compatible with the heat-treatment cycles of sintering and hardening. High-performance PM steel components usually need to be hardened after sintering, either by a quenching and tempering, or case-hardening treatment. In the increasingly popular and cost-effective sinter-furnace hardening process the benefits have been steadily improved by the adoption of accelerated cooling systems in sintering furnaces, enabling the levels of key alloying elements to be reduced. However, the successful application of sinter-hardening to produce finished PM components that meet the customers' specifications requires an understanding of the factors governing the response of low-alloy steel to heat treatment. The conundrum is that to achieve satisfactory hardening of a component in a given cross-section one needs either a sufficiently rapid cooling rate through the critical temperature range, or sufficient dissolved alloy content to provide the hardenability. With PM there is the added influence of density and the question that key alloying elements like nickel, chromium and manganese reduce the compressibility if present as pre-alloyed elements in the ferrous powder and are generally less effective as admixed constituents. Then there is the question of cost: juggling these inter-related factors for high-strength performance parts has provided a serious challenge for powder producers and parts fabricators alike.
The basic element is the steel characteristic known by the term “hardenability”. With PM steels the hardenability is affected by the density in addition to the chemistry and austenitising conditions. The classic test for determining wrought steel hardenability is the Jominy End-Quench Test where the depth of hardening in a standardised cylindrical test-piece is measured after quenching from one end with a water-jet. In a scholarly investigation, Peter Sokolowski and Bruce Lindsley of Hoeganaes Corporation measured the hardenability of a series of PM steels based on available commercial sinter-hardenable steel powders with the addition of 1% or 2% of copper powder and 0.6% or 0.9% graphite (Table 1). The hardenability and microstructure results showed the expected correlations with chemistry (Figure 1). In addition, the influence of austenitising temperature was studied. Increasing the austenitising temperature from 845° to 950° made some alloys more hardenable, particularly the steels with higher molybdenum contents. Further investigation showed that this effect was likely due to the increased dissolution of (Fe, Mo) carbides, providing more molybdenum content in the austenite.
Table 1. Hardenability of PM steels: Nominal compositions of base pre-alloyed powders studied by Sokolowski & Lindsley.
MPIF Designation |
Mo% |
Ni% |
Mn% |
Fe% |
|
Ancorsteel*2000 |
FL-4200 |
0.6 |
0.5 |
0.25 |
Bal. |
Ancorsteel 4600V |
FL-4600 |
0.55 |
1.8 |
0.15 |
Bal. |
Ancorsteel 721 SH |
–– |
0.9 |
0.5 |
0.4 |
Bal. |
Ancorsteel 737 SH |
FL-4800 |
1.25 |
1.4 |
0.4 |
Bal. |
Ancorsteel 150 HP |
FL-4900 |
1.5 |
– |
0.1 |
Bal. |
* Ancorsteel is a registered trademark of Hoeganaes Corporation.
While the object of the study was to aid parts producers in the selection of suitable alloy systems for sinter-hardening applications, the authors were surprised that some of their results conflicted with data published in MPIF Standard 35, which used a lower austenitising temperature. It was suggested that the temperature used in the MPIF evaluation was insufficient to completely austenitise alloys with combinations of high molybdenum and high carbon. While recognising that leaner alloy systems raised questions about reduced hardenability, in a companion paper, Bruce Lindsley looked for quantitative answers. He compared the hardenability of leaner alloy steel powders by studying transformations during heat-treatment by dilatometry and microstructure. He used the results to construct “continuous sinter-cooling transformation diagrams” for a series of low alloy steel compositions based on recently-introduced lean alloy steel powders Ancorsteel 721SH and Ancorsteel 4300 and 4300L (Table 2). He compared the results with those obtained for three of the standard compositions for sinter-hardening: FL4608, FLC2-4808 and FLNC-4408. The judicious selection of alloy elements showed that the lower Mo Ancorsteel 4300L performed well versus the earlier Ancorsteel 4300 version and does not require the admixing of copper to boost the hardenability.
Table 2. Compositions of PM Alloy Steels Tested by Lindsley.
Alloy |
C |
Mn |
Si |
Ni |
Cr |
Mo |
Cu |
|
Ancorsteel 721 SH |
1 |
0.80 |
0.4 |
– |
0.5 |
– |
0.9 |
– |
Ancorsteel 721 SH |
2 |
0.60 |
0.4 |
– |
0.5 |
– |
0.9 |
1.0 |
Ancorsteel 721 SH |
3 |
0.78 |
0.4 |
– |
0.5 |
– |
0.9 |
2.0 |
Ancorsteel 4300L |
4 |
0.64 |
0.1 |
0.6 |
1.0 |
1.0 |
0.3 |
– |
FL-4800 |
– |
– |
0.4 |
– |
1.4 |
– |
1.2 |
– |
FL-4600 |
– |
– |
0.1 |
– |
1.8 |
– |
0.5 |
– |
Ancorsteel 4300 |
– |
– |
0.1 |
0.6 |
1.0 |
1.0 |
0.8 |
– |
FLNC-4408 |
– |
0.75 |
0.1 |
– |
2.0 |
– |
0.8 |
1.5 |
Ancorsteel 4300L is also more tolerant of high carbon levels than the original 4300, so that the use of increased graphite content with Ancorsteel 4300L more than offset the reduced molybdenum level with respect to hardenability. However, a fully-martensitic microstructure was not achieved with Ancorsteel 4300L for any of the cooling rates tested. For this reason, the lean 4300L alloy was not recommended for applications where a nearly 100% martensite structure was required. On the other hand, a significant percentage of martensite (40% to 75%) was easily obtainable by sinter-hardening, and provided good mechanical properties over a range of cooling rates.
The latest powder producer to introduce new products for sinter-hardening is Pometon SpA of Italy. Properties developed in sinter-hardening tests on new PM steels based on proprietary Cr-Mo-Ni steel powders from Pometon SpA were described by Stefano Saccarola (Pometon) and in parallel presentations co-authored by Professor Francisco Castro and colleagues at CEIT and Tecnun (Spain). Structures and properties of Cr–Mo-Ni steels, with or without copper additions suitable for either normal or accelerated cooling were studied for optimisation of compositions. Tests showed that discs of the new alloy, 35 mm in diameter and 20 mm thick were fully martensitic after cooling at 0.45°/second.
Alongside the efforts of major powder producers to fine-tune their established pre-alloyed powder grades, there have been several attempts in recent years to find a way to employ the strong hardenability contribution of manganese. Manganese has been used successfully in wrought low-alloy steels for many decades. Unfortunately, in ferrous powders pre-alloyed manganese reduces the compressibility significantly and so has been used only in minor quantities, typically less than 0.5%.
Elemental manganese powder, on the other hand, is subject to several disadvantages when used as an admixed component in the PM process. It oxidises easily, hydrolyses, and is hard to reduce under conventional sintering conditions. The high volatility of heated manganese also results in serious losses and furnace contamination. Attempts to avoid these formidable problems by the use of master-alloys, ferro-manganese or manganese-containing mixed carbides have encountered other problems stemming from tool abrasion and patent restrictions.
In continuing work with his proprietary chemical treatment techniques, Dennis Hammond (Apex Advanced Technologies LLC) showed how he had overcome the above difficulties by applying a protective coating to manganese powder particles before admixing. This (patent-pending) approach allows high enough levels of manganese to be effective in providing hardenability without adversely affecting compacted density. The resulting premixes with plain iron or pre-alloyed Cr–Mo steel powders could be sintered or sinter-hardened in conventional furnaces. The manganese metal powder is coated with a hydrophobic coating in an inert atmosphere. The treated manganese is mixed with graphite and Apex SuperLube® special lubricant in a screened additive “master-batch” that enables high densities to be achieved in compaction. A pre-calculated density range is provided to achieve semi-hydrostatic conditions. Densification is maximised by the creation of a semi-hydrostatic state during compaction that Hammond has described in previous presentations. This condition is also critical in the protection of the elemental manganese in the early (wettest) stages of sintering.
In the latest test illustrations, blending, compacting and sintering were conducted in standard commercial equipment. Hammond chose four compositions to suit different categories of applications: (1) case-hardenable, (2) general purpose, (3) sinter-hardenable and (4) high-performance sinter-hardenable. In each composition the major alloying element was manganese at the 1% level (Table 3). These materials were pressed into slugs 3.5 inches in diameter by 0.9 inches thick, compacted to 7.1-7.2 g/cm3, and sintered under
a variety of process conditions. These included slow and fast cooling in a Sinterite belt furnace, and rapid cooling from 2350°F in an Abbott pusher furnace. Post-sintering heat treatments were also performed at 1550°F/40 minutes in an atmosphere with a carbon potential of 0.85% followed by tempering at 350/400°F.
Table 3. Manganese Low-Alloy PM Steel Compositions (After Hammond).
Manganese % |
Chromium % |
Molybdenum % |
Carbon %* |
|
Case-hardenable |
1.0 |
–– |
–– |
0.27/0.35 |
General Purpose |
1.0 |
0.5 |
0.07 |
0.53/0.58 |
Sinter-hardenable |
1.0 |
0.5 |
0.07 |
0.70/0.75 |
High-Performance |
1.0 |
0.75 |
0.34 |
0.68/0.71 |
Sinter-hardenable |
* As sintered
Hammond showed results for density, dimensional change, hardness, impact values and TRS for seven different treatments. It is not possible to summarise all these results here, so a selection of data for the sinter-hardening compositions are shown in Table 4. Hammond concluded that through its hardenability factor, manganese was the primary contributor to desirable properties, claiming higher than normal hardness and impact property values for both case-hardening and sinter-hardening. Properties generally improved with sintering time and temperature, while strength (TRS) values depended on carbon levels. Carbon loss during sintering was normal (0.05%) for the plain manganese compositions and a bit higher (0.12-0.2%) with the presence of higher chromium contents. Hammond reported several successes in introducing his manganese master batch for use in PM part fabrication with commercially-available iron and steel powder grades and showed an example of one item already in production.
Table 4.
Properties of Sinter-hardened 1% Manganese PM Steels (After Hammond)
Treatment |
Density (g/cm3) |
Hardness (HRB) |
TRS (MPa) |
Impact Value (J) |
2050°F/30 min./Fast cool |
7.18 |
101 |
847 |
8.3 |
2350°F/30 min./Fast cool |
7.26 |
107 |
1074 |
21 |
Material: High-performance Sinter-hardenable !% Mn, 0.75% Cr, 0.34% Mo (0.68/0.73% C) |
||||
Treatment |
Density (g/cm3) |
Hardness (HRB) |
TRS (MPa) |
Impact Value (J) |
2050°F/30 min./Fast cool |
7.11 |
114 |
800 |
7 |
2350°F/30 min./Fast cool |
7.2 |
117 |
870 |
11 |
In conclusion, the pressure to scale back the employment of expensive alloy elements needs to be pursued with an eye on the realities of alloy steel metallurgy. This is especially true for high-performance components that have come to be manufactured with the use of sinter-hardening as a cost-reducing process step to meet stringent hardness and toughness requirements.
In the past, sinter-hardening has been applied with materials consisting of combinations of pre-alloyed and admixed alloy elements, where adequate hardenability was easily ensured by piling on the total alloy content. However, cost-cutting by reducing the use of expensive alloys has to be done with the knowledge of the relative contributions of composition and density, as well as sintering (austenitising) temperature and cooling conditions. The message from these latest investigations emphasises the care needed in making the right choices.
The exaggerated volatility in energy and raw materials prices over the last few years has resulted in continuing problems for component manufacturers in all industry sectors. For ferrous powder producers as well as PM parts makers, “riding the alloy price tiger” has caused a flurry of development activity aimed at finding lower-cost solutions of various kinds, particularly for high-performance applications. As expected, there was no shortage of presentations at the MPIF's PowderMet2009 conference in Las Vegas discussing various aspects of leaner alloy systems for high-strength PM steel components.
Much of the debate so far has ranged around finding satisfactory alternatives to low-alloy steel compositions with high levels of the traditional elements nickel, copper and molybdenum that saw extraordinary price rises up to the current recession. As is well known, these alloy systems have been popular for a long time because of their ease of application in the conventional PM process. Nickel and copper are typically used in elemental admixed form, while molybdenum has found increased application as a fully pre-alloyed constituent because of its strong contribution to hardenability and minimal impact on compressibility. All three elements have the advantage of easily-reducible oxides which makes them very compatible with the heat-treatment cycles of sintering and hardening. High-performance PM steel components usually need to be hardened after sintering, either by a quenching and tempering, or case-hardening treatment. In the increasingly popular and cost-effective sinter-furnace hardening process the benefits have been steadily improved by the adoption of accelerated cooling systems in sintering furnaces, enabling the levels of key alloying elements to be reduced. However, the successful application of sinter-hardening to produce finished PM components that meet the customers' specifications requires an understanding of the factors governing the response of low-alloy steel to heat treatment. The conundrum is that to achieve satisfactory hardening of a component in a given cross-section one needs either a sufficiently rapid cooling rate through the critical temperature range, or sufficient dissolved alloy content to provide the hardenability. With PM there is the added influence of density and the question that key alloying elements like nickel, chromium and manganese reduce the compressibility if present as pre-alloyed elements in the ferrous powder and are generally less effective as admixed constituents. Then there is the question of cost: juggling these inter-related factors for high-strength performance parts has provided a serious challenge for powder producers and parts fabricators alike.
The basic element is the steel characteristic known by the term “hardenability”. With PM steels the hardenability is affected by the density in addition to the chemistry and austenitising conditions. The classic test for determining wrought steel hardenability is the Jominy End-Quench Test where the depth of hardening in a standardised cylindrical test-piece is measured after quenching from one end with a water-jet. In a scholarly investigation, Peter Sokolowski and Bruce Lindsley of Hoeganaes Corporation measured the hardenability of a series of PM steels based on available commercial sinter-hardenable steel powders with the addition of 1% or 2% of copper powder and 0.6% or 0.9% graphite (Table 1). The hardenability and microstructure results showed the expected correlations with chemistry (Figure 1). In addition, the influence of austenitising temperature was studied. Increasing the austenitising temperature from 845° to 950° made some alloys more hardenable, particularly the steels with higher molybdenum contents. Further investigation showed that this effect was likely due to the increased dissolution of (Fe, Mo) carbides, providing more molybdenum content in the austenite.
Table 1. Hardenability of PM steels: Nominal compositions of base pre-alloyed powders studied by Sokolowski & Lindsley.
MPIF Designation |
Mo% |
Ni% |
Mn% |
Fe% |
|
Ancorsteel*2000 |
FL-4200 |
0.6 |
0.5 |
0.25 |
Bal. |
Ancorsteel 4600V |
FL-4600 |
0.55 |
1.8 |
0.15 |
Bal. |
Ancorsteel 721 SH |
–– |
0.9 |
0.5 |
0.4 |
Bal. |
Ancorsteel 737 SH |
FL-4800 |
1.25 |
1.4 |
0.4 |
Bal. |
Ancorsteel 150 HP |
FL-4900 |
1.5 |
– |
0.1 |
Bal. |
* Ancorsteel is a registered trademark of Hoeganaes Corporation.
While the object of the study was to aid parts producers in the selection of suitable alloy systems for sinter-hardening applications, the authors were surprised that some of their results conflicted with data published in MPIF Standard 35, which used a lower austenitising temperature. It was suggested that the temperature used in the MPIF evaluation was insufficient to completely austenitise alloys with combinations of high molybdenum and high carbon. While recognising that leaner alloy systems raised questions about reduced hardenability, in a companion paper, Bruce Lindsley looked for quantitative answers. He compared the hardenability of leaner alloy steel powders by studying transformations during heat-treatment by dilatometry and microstructure. He used the results to construct “continuous sinter-cooling transformation diagrams” for a series of low alloy steel compositions based on recently-introduced lean alloy steel powders Ancorsteel 721SH and Ancorsteel 4300 and 4300L (Table 2). He compared the results with those obtained for three of the standard compositions for sinter-hardening: FL4608, FLC2-4808 and FLNC-4408. The judicious selection of alloy elements showed that the lower Mo Ancorsteel 4300L performed well versus the earlier Ancorsteel 4300 version and does not require the admixing of copper to boost the hardenability.
Table 2. Compositions of PM Alloy Steels Tested by Lindsley.
Alloy |
C |
Mn |
Si |
Ni |
Cr |
Mo |
Cu |
|
Ancorsteel 721 SH |
1 |
0.80 |
0.4 |
– |
0.5 |
– |
0.9 |
– |
Ancorsteel 721 SH |
2 |
0.60 |
0.4 |
– |
0.5 |
– |
0.9 |
1.0 |
Ancorsteel 721 SH |
3 |
0.78 |
0.4 |
– |
0.5 |
– |
0.9 |
2.0 |
Ancorsteel 4300L |
4 |
0.64 |
0.1 |
0.6 |
1.0 |
1.0 |
0.3 |
– |
FL-4800 |
– |
– |
0.4 |
– |
1.4 |
– |
1.2 |
– |
FL-4600 |
– |
– |
0.1 |
– |
1.8 |
– |
0.5 |
– |
Ancorsteel 4300 |
– |
– |
0.1 |
0.6 |
1.0 |
1.0 |
0.8 |
– |
FLNC-4408 |
– |
0.75 |
0.1 |
– |
2.0 |
– |
0.8 |
1.5 |
Ancorsteel 4300L is also more tolerant of high carbon levels than the original 4300, so that the use of increased graphite content with Ancorsteel 4300L more than offset the reduced molybdenum level with respect to hardenability. However, a fully-martensitic microstructure was not achieved with Ancorsteel 4300L for any of the cooling rates tested. For this reason, the lean 4300L alloy was not recommended for applications where a nearly 100% martensite structure was required. On the other hand, a significant percentage of martensite (40% to 75%) was easily obtainable by sinter-hardening, and provided good mechanical properties over a range of cooling rates.
The latest powder producer to introduce new products for sinter-hardening is Pometon SpA of Italy. Properties developed in sinter-hardening tests on new PM steels based on proprietary Cr-Mo-Ni steel powders from Pometon SpA were described by Stefano Saccarola (Pometon) and in parallel presentations co-authored by Professor Francisco Castro and colleagues at CEIT and Tecnun (Spain). Structures and properties of Cr–Mo-Ni steels, with or without copper additions suitable for either normal or accelerated cooling were studied for optimisation of compositions. Tests showed that discs of the new alloy, 35 mm in diameter and 20 mm thick were fully martensitic after cooling at 0.45°/second.
Alongside the efforts of major powder producers to fine-tune their established pre-alloyed powder grades, there have been several attempts in recent years to find a way to employ the strong hardenability contribution of manganese. Manganese has been used successfully in wrought low-alloy steels for many decades. Unfortunately, in ferrous powders pre-alloyed manganese reduces the compressibility significantly and so has been used only in minor quantities, typically less than 0.5%.
Elemental manganese powder, on the other hand, is subject to several disadvantages when used as an admixed component in the PM process. It oxidises easily, hydrolyses, and is hard to reduce under conventional sintering conditions. The high volatility of heated manganese also results in serious losses and furnace contamination. Attempts to avoid these formidable problems by the use of master-alloys, ferro-manganese or manganese-containing mixed carbides have encountered other problems stemming from tool abrasion and patent restrictions.
In continuing work with his proprietary chemical treatment techniques, Dennis Hammond (Apex Advanced Technologies LLC) showed how he had overcome the above difficulties by applying a protective coating to manganese powder particles before admixing. This (patent-pending) approach allows high enough levels of manganese to be effective in providing hardenability without adversely affecting compacted density. The resulting premixes with plain iron or pre-alloyed Cr–Mo steel powders could be sintered or sinter-hardened in conventional furnaces. The manganese metal powder is coated with a hydrophobic coating in an inert atmosphere. The treated manganese is mixed with graphite and Apex SuperLube® special lubricant in a screened additive “master-batch” that enables high densities to be achieved in compaction. A pre-calculated density range is provided to achieve semi-hydrostatic conditions. Densification is maximised by the creation of a semi-hydrostatic state during compaction that Hammond has described in previous presentations. This condition is also critical in the protection of the elemental manganese in the early (wettest) stages of sintering.
In the latest test illustrations, blending, compacting and sintering were conducted in standard commercial equipment. Hammond chose four compositions to suit different categories of applications: (1) case-hardenable, (2) general purpose, (3) sinter-hardenable and (4) high-performance sinter-hardenable. In each composition the major alloying element was manganese at the 1% level (Table 3). These materials were pressed into slugs 3.5 inches in diameter by 0.9 inches thick, compacted to 7.1-7.2 g/cm3, and sintered under a variety of process conditions. These included slow and fast cooling in a Sinterite belt furnace, and rapid cooling from 2350°F in an Abbott pusher furnace. Post-sintering heat treatments were also performed at 1550°F/40 minutes in an atmosphere with a carbon potential of 0.85% followed by tempering at 350/400°F.
Table 3. Manganese Low-Alloy PM Steel Compositions (After Hammond).
Manganese % |
Chromium % |
Molybdenum % |
Carbon %* |
|
Case-hardenable |
1.0 |
–– |
–– |
0.27/0.35 |
General Purpose |
1.0 |
0.5 |
0.07 |
0.53/0.58 |
Sinter-hardenable |
1.0 |
0.5 |
0.07 |
0.70/0.75 |
High-Performance |
1.0 |
0.75 |
0.34 |
0.68/0.71 |
Sinter-hardenable |
* As sintered
Hammond showed results for density, dimensional change, hardness, impact values and TRS for seven different treatments. It is not possible to summarise all these results here, so a selection of data for the sinter-hardening compositions are shown in Table 4. Hammond concluded that through its hardenability factor, manganese was the primary contributor to desirable properties, claiming higher than normal hardness and impact property values for both case-hardening and sinter-hardening. Properties generally improved with sintering time and temperature, while strength (TRS) values depended on carbon levels. Carbon loss during sintering was normal (0.05%) for the plain manganese compositions and a bit higher (0.12-0.2%) with the presence of higher chromium contents. Hammond reported several successes in introducing his manganese master batch for use in PM part fabrication with commercially-available iron and steel powder grades and showed an example of one item already in production.
Table 4.
Properties of Sinter-hardened 1% Manganese PM Steels (After Hammond)
Treatment |
Density (g/cm3) |
Hardness (HRB) |
TRS (MPa) |
Impact Value (J) |
2050°F/30 min./Fast cool |
7.18 |
101 |
847 |
8.3 |
2350°F/30 min./Fast cool |
7.26 |
107 |
1074 |
21 |
Material: High-performance Sinter-hardenable !% Mn, 0.75% Cr, 0.34% Mo (0.68/0.73% C) |
||||
Treatment |
Density (g/cm3) |
Hardness (HRB) |
TRS (MPa) |
Impact Value (J) |
2050°F/30 min./Fast cool |
7.11 |
114 |
800 |
7 |
2350°F/30 min./Fast cool |
7.2 |
117 |
870 |
11 |
In conclusion, the pressure to scale back the employment of expensive alloy elements needs to be pursued with an eye on the realities of alloy steel metallurgy. This is especially true for high-performance components that have come to be manufactured with the use of sinter-hardening as a cost-reducing process step to meet stringent hardness and toughness requirements.
In the past, sinter-hardening has been applied with materials consisting of combinations of pre-alloyed and admixed alloy elements, where adequate hardenability was easily ensured by piling on the total alloy content. However, cost-cutting by reducing the use of expensive alloys has to be done with the knowledge of the relative contributions of composition and density, as well as sintering (austenitising) temperature and cooling conditions. The message from these latest investigations emphasises the care needed in making the right choices.