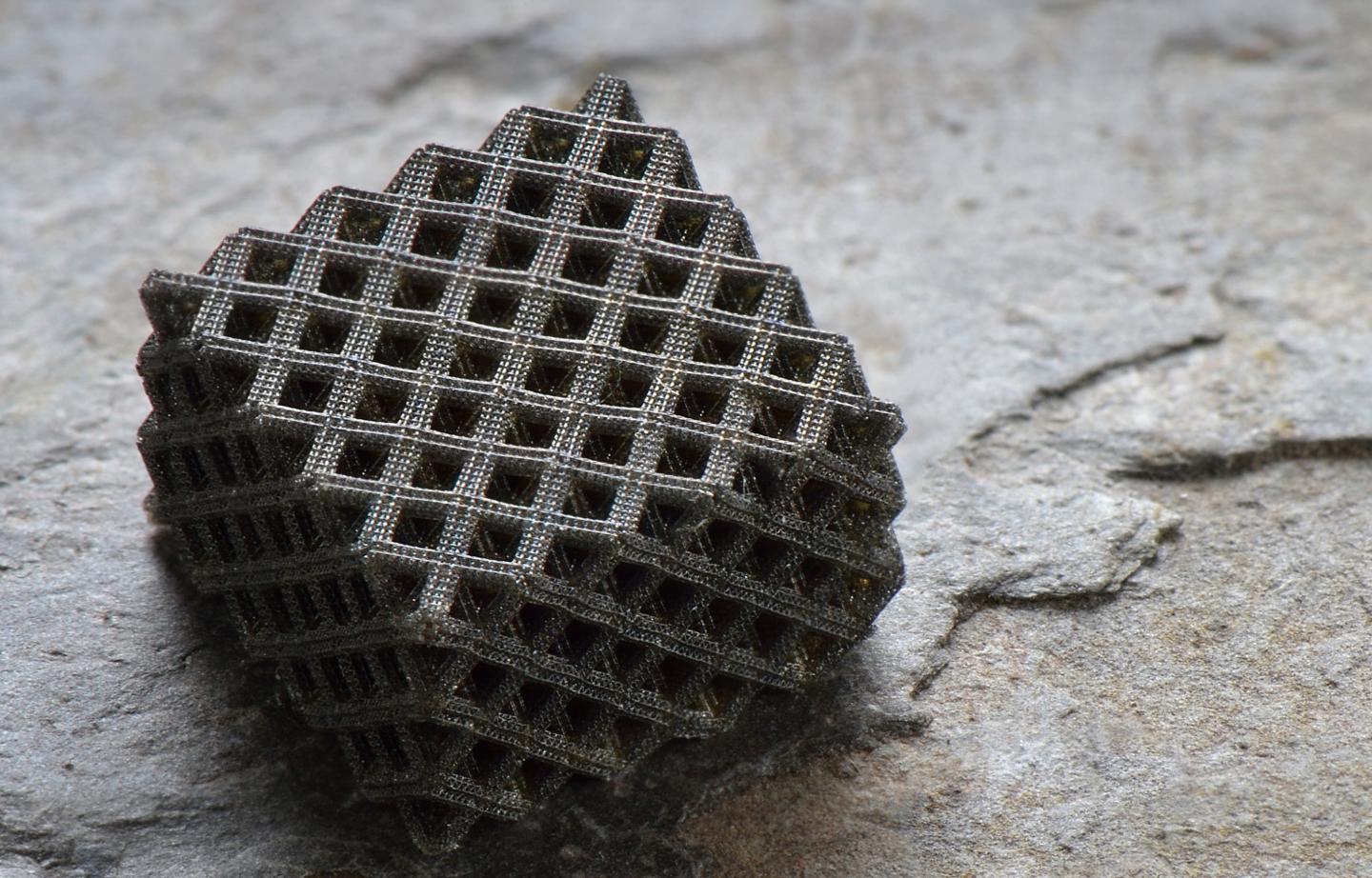
For years, scientists and engineers have synthesized nanoscale materials to take advantage of their mechanical, optical and energy properties, but efforts to scale these materials up to larger sizes have simply diminished their performance and structural integrity. Materials that can be produced at the nanoscale, such as graphene sheets, can be 100 times stronger than steel. But trying to upsize these materials in three dimensions degrades their strength by eight orders of magnitude – in other words, they become 100 million times less strong.
Now, researchers led by Xiaoyu ‘Rayne’ Zheng, an assistant professor of mechanical engineering at Virginia Tech, have published a paper in Nature Materials that describes a new process for creating lightweight, strong and super-elastic 3D printed metallic nanostructured materials with unprecedented scalability. This new process offers a full seven orders of magnitude of control over arbitrary 3D architectures.
Using this process, the researchers have already created multiscale metallic materials that display super elasticity because of their designed hierarchical 3D architectural arrangement and nanoscale hollow tubes. The resultant materials boast a 400% increase in tensile elasticity over conventional lightweight metals and ceramic foams.
The approach, which produces multiple levels of 3D hierarchical lattices with nanoscale features, could prove of use anywhere there's a need for a combination of stiffness, strength, low weight and high flexibility. This could include structures to be deployed in space, flexible armors, and lightweight vehicles and batteries, opening the door for applications in aerospace, military and automotive industries.
Natural materials, such as trabecular bone and the toes of geckoes, have evolved multiple levels of 3D architectures, spanning from the nanoscale to the macroscale. Until now, scientists hadn’t managed to achieve this delicate level of control over the structural features of synthetic materials.
"Creating 3D hierarchical micro features across the entire seven orders of magnitude in structural bandwidth in products is unprecedented," said Zheng, the lead author of the study and the research team leader. "Assembling nanoscale features into billets of materials through multi-leveled 3D architectures, you begin to see a variety of programmed mechanical properties such as minimal weight, maximum strength and super elasticity at centimeter scales."
The process that Zheng and his collaborators use to create the materials represents an innovation in a digital light 3D printing technique. This innovation overcomes current trade-offs between high resolution and build volume, a major limitation in the scalability of current 3D printed microlattices and nanolattices.
"The increased elasticity and flexibility obtained through the new process and design come without incorporating soft polymers, thereby making the metallic materials suitable as flexible sensors and electronics in harsh environments, where chemical and temperature resistance are required," Zheng added.
One of the great opportunities offered by this new technique is the ability to produce multi-functional inorganic materials such as metals and ceramics for photonic and energy harvesting. With multi-leveled hierarchical lattices, more surface area is available to collect photons of light as they enter the structure from all directions. This means that rather than collect photons just on the outer surface of the material, as with traditional photovoltaic panels, they can also be collected inside the lattice structure.
Besides Zheng, team members included Virginia Tech graduate research students Huachen Cui and Da Chen from Zheng's group, and colleagues from Lawrence Livermore National Laboratory.
This story is adapted from material from Virginia Tech, with editorial changes made by Materials Today. The views expressed in this article do not necessarily represent those of Elsevier. Link to original source.